Over the past 115 years, physicists have discovered that almost everything material, including rocks, rain, sun and sunlight, ocean waves and radio waves, can be described in terms of particles (and their corresponding fields). Experiments have discovered a variety of types of particles, which today seem to us elementary (that is, they do not consist of more elementary particles). All the complex diversity of our familiar world consists of a small set of such particles. The remaining particles are fleeting, they disintegrate so quickly that under normal conditions we do not meet them. But they can keep the keys to the secrets of the universe that remain inaccessible to us.
In this article you will find a small overview of the current understanding of particles and their organization into classes. Something like a periodic table of particles with a couple of dirty tricks. In addition, you will learn what the Higgs field does with particles and its critical role in the life of the Universe.
Our current understanding, together with the simplest hypotheses about the particle and the Higgs field, is reduced to a set of equations called the Standard Model of Particle Physics, or simply the Standard Model. Elementary particles in the Standard Model historically have very strange names, as well as a large scatter of the masses. In fig. one:
• I painted heavier particles on the top, and light ones on the bottom. By my logic, massless particles are the minimum level, and there is no upper limit for the mass of particles. That is, there is a hard floor below, and only sky above.
• Instead of masses, I give equivalent mass-energies (E = mc
2 ), which are usually used by specialists in particle physics. It is easier to follow the energy, which does not disappear and does not appear, than to monitor the mass of particles, which can change in certain processes, for example,
during decay . The unit of GeV is approximately equal to the mass-energy of the lightest atom, hydrogen.
• I identified three classes of particles: charged leptons (blue disks), neutrinos (black disks), and quarks (red disks). Quarks are usually divided into two classes, upper and lower, differing only in electric charge. The importance of such a classification will become clear later.
• In the rectangles, I indicated three interactions with their particle carriers. The fourth interaction, gravity, I excluded, so as not to litter the picture.
• The Higgs field (or something that plays its role) in nature is on average non-zero. I marked it through the green background.

Fig. one
What are these particles? All of them have antiparticles, but for brevity, I omit them. Let's quickly go over the structure of matter, sorting it out until we get to the desired level.
• Atoms whose radius is about a billion times smaller than your head are composed of electrons and atomic nuclei.
• Atoms absorb and emit particles of light, photons. This happens due to the electromagnetic interaction carried by the photon (that is, when electromagnetism is working, photons are always present).
• Atomic nuclei consist of protons and neutrons, 100,000 smaller than the atom itself, and consisting mostly of upper and lower quarks (and antiquarks) and gluons.
• Protons and neutrons do not fall apart, and are also retained inside the nucleus, due to the strong interaction carried by 8 types of gluons.
• The sun shines, and some atomic nuclei decay due to the transformation processes of quarks of one type into quarks of another type. In this case, electrons and neutrinos are emitted, these particles emanate directly from the center of the sun.
• This transformation of quarks and the emission of neutrinos is due to the weak interaction carried by the particles W
+ , W
- and Z
0 .
• The last known interaction is gravity, which is supposed to carry the graviton. Due to the amazing weakness of gravity, this particle is very difficult to detect.
Virtually all aspects of our world are determined by these particles. But there are others. The electron, neutrino-1, the upper quark and the lower quark are called one “generation” of particles - in this case, by generation we mean approximately the same as in the family tree. There are two heavier generations, each of which has weighted copies of these four particles.
• The second generation consists of a muon, a neutrino-2, an enchanted quark and a strange quark.
• The third generation consists of tau, neutrino-3, t-quark and b-quark.
The structure of generations divides these particles into horizontal layers. They can also be divided vertically into the classes I mentioned: people often talk about "electron-type particles" or "charged leptons", meaning electron, muon and tau, talk about "neutrino" in general, and divide quarks into "upper" (upper, enchanted, t) and "lower" (lower, strange, b).
You may wonder why neutrinos have such boring names compared to other particles. We called them differently, but over the past 20 years we have learned a lot about them, and still continue to learn. Maybe when the dust settles, we will give them new names.
We know little about the Higgs particle, but we will learn more soon.
Let's look closely at different masses. They have not only a huge spread, but there is no any explicit system. Here are some notes about the masses, starting with the lightest particles:
• Photon and graviton probably do not have mass - their mass must be surprisingly small for the observed intergalactic magnetic fields and huge structures of the Universe.
• Gluons do not have mass, as far as it makes sense - they spend their lives in captivity inside such hadrons as protons, and measuring their mass is not straightforward.
• Theorists have long argued about the presence of mass in neutrinos. Experiments of the last decade have resolved this dispute (although due to the fact that the evidence obtained is indirect, there is still room for maneuver). The neutrino masses are very small, the heaviest of them are at least a billion times lighter than the lightest atom (hydrogen), and the mass of the lightest is even smaller.
• The masses of the remaining particles are known. An electron is about 1800 times lighter than hydrogen, a t-quark is about 400,000 times heavier than an electron, and only a few percent lighter than a gold atom. The mass of W and Z particles is approximately half the mass of the t-quark.
• All high-mass particles have it due to interaction with the Higgs field. Neutrinos can receive mass indirectly, but the Higgs field plays an important role for them too. I noted this fact through the green frames of varying thickness at the disks, denoting particles.
• Higgs particle mass-energy - 125 GeV
In fig. 2 I grouped particles and interactions differently.
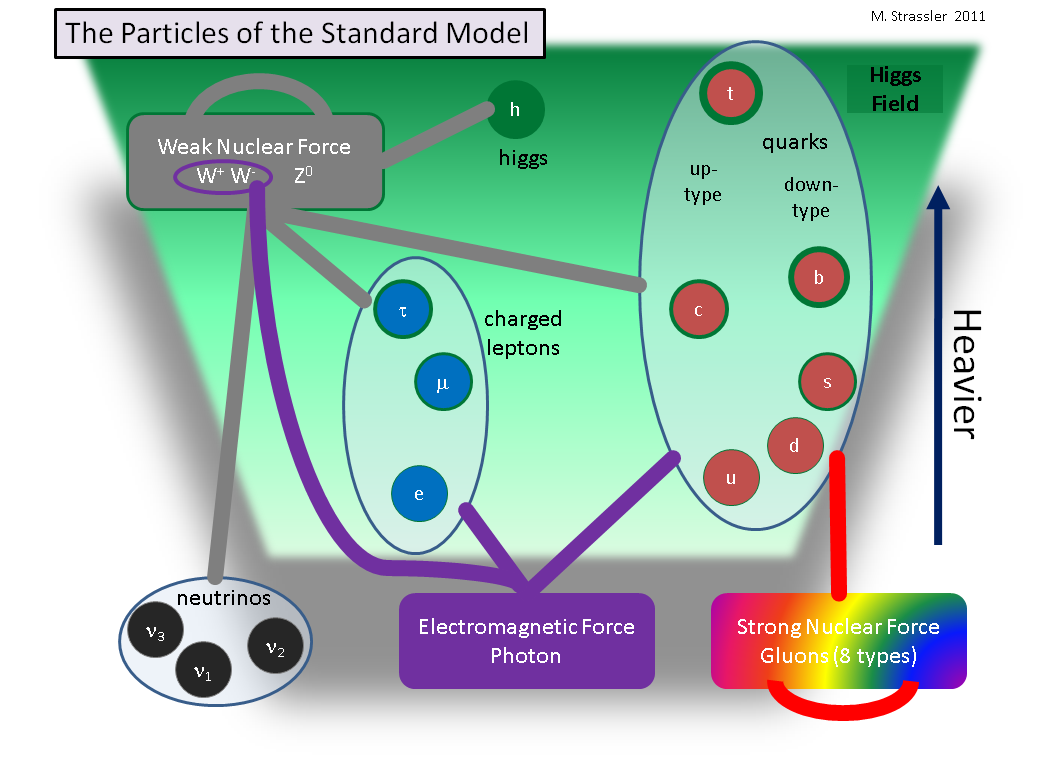
Fig. 2
The figure shows which particles directly affect which. I drew lines between all types of particles that directly interact with each other. What is interesting to note:
• Nothing of what is often called matter particles — charged leptons, neutrinos, or quarks — does not interact with each other.
• Particles of matter interact directly only with particles that carry interactions!
This explains why the interaction carriers are called that way. When an electron in an atom interacts with an upper quark in an atomic nucleus, it does not do it directly. The electron directly interacts with the photon, the quark interacts with the photon, and as a result (it is quite complex and unintuitive) it turns out that the electron is attracted to the quark, and vice versa. In the same way, the interaction between two quarks is obtained indirectly, and comes from the direct interaction of quarks with gluons. All known interactions between particles of matter occur indirectly, they involve interaction carriers. When you open the door, the photons work.
The figure also highlights several important properties of interactions and particle classes:
• All particles of a certain class obey one interaction - this is what determines their belonging to a class. Neutrinos feel only weak interaction. Only quarks and gluons feel strong interaction.
• Curved lines indicate that some of the interaction carriers directly interact with themselves or with other carriers. Gluons interact with themselves, but the photon does not interact with itself (at least, not directly).
• In a sense, the Higgs particle is also a carrier of the interaction. But this is a special case. The stronger the effect of the Higgs interaction on the particle, the greater the mass of this particle in the non-zero Higgs field. (This statement is true for known particles, but it may turn out to be wrong for those not yet open). I marked this with the gradient of the green field, which becomes darker from above, which means an increase in the effect for heavy particles. In the same way, the Higgs particle interacts more with heavy particles than with light ones.
This world looks awfully strange, but whether you like it or not, it’s ours. You can see some roughly marked schemes, but still there is no clear organization. Disorganization is somehow connected with the Higgs field (s).