In recent years, scientists have tried various unusual inks for printing on 3D printers. Some inks were made of heat-sensitive polymers and were needed to print objects that change shape under the influence of heat. Others printed light-sensitive polymeric structures that shrink and stretch in response to streams of light. Experts from the Massachusetts Institute of Technology (MIT)
have made a "live" tattoo. The term "tattoo" is not entirely accurate. The pattern of bacteria does not impale on the skin, but is glued with a liquid gel after layer-by-layer printing, and the bacteria live inside the printed walls of the pattern while there are food resources. Bacteria can react to external stimuli by changing their permeability, vibrating or changing their color (more correct specialized terms are given below) and thereby signaling the owner about health or environmental problems or transmitting information to a certain device. In the example shown, the “live sensor” used the fluorescence mechanism (glowed).
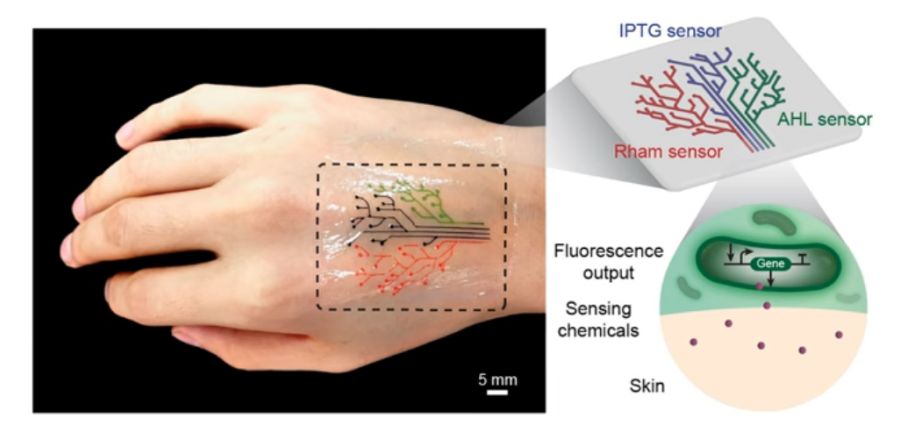
The research team, led by Professor Xuanhe Zhao and Associate Professor Timothy Lou, demonstrated her technique to reporters by printing a “live tattoo” - a thin, transparent overlay (patch) in the shape of a tree. Each of the 3 branches of the tree was saturated with cells sensitive to a particular chemical or molecular compound. Then attached to a transparent elastomeric layer and checked the effect of the patch on the volunteer's wrist. Several chemical compounds were applied to the skin. When the patch was applied to the skin, which was exposed to different compounds, the desired areas of the tree were lit in response.
The sensor worked for several hours and during this time each of the 3 “branches” of the sensor was lit when the bacteria sensed the corresponding chemical stimuli. The color change was associated with the launch of the fluorescent proteins inside the bacterial cells.
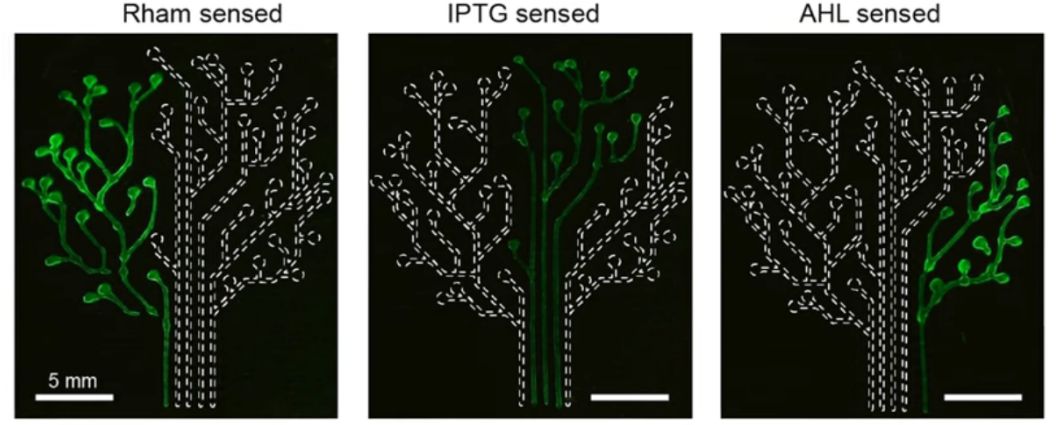
The pattern was printed on the basis of a standard three-dimensional printer, but in combination with devices that were modified by themselves. To print on a 3D printer, it was necessary to genetically modify the walls of bacteria, bringing them to a density capable of withstanding the pressure of the jet from the printer during 3D printing. Mammalian lipid cells, previously used in other studies, simply burst from the stress of typing. It was necessary to further increase the survival of the bacteria themselves. The researchers conducted a screening test to determine the type of hydrogel that would best accept bacterial cells. After an extensive search, the best combination was a hydrogel with pluronic acid as the most compatible material. After printing, the pattern was hardened under ultraviolet radiation and became a “smart” sensor sticker (patches).
The researchers also created bacteria to communicate with each other; for example, they programmed some cells for lighting only when they receive a certain signal from another cell. To test this type of connection in a three-dimensional structure, they printed a thin sheet of hydrogel filaments with “inputs” (signal generating) bacteria and a layer of threads laid on them with “outputs” (signal receiving) bacteria. Scientists have discovered that the output fibers are illuminated only when they receive input signals from the relevant bacteria. In the future, humanity will be able to use this technique for the work of “living computers” - structures with several types of cells that communicate with each other, transmitting signals back and forth, like transistors on a microchip.
This is a distant prospect, but the authors of the study expect to print live wearable computers someday. Currently, the application will be individual sensors in the form of flexible stickers. The plans also include the creation of surgical implant factories that produce useful compounds inside the person, such as glucose. "We can use bacterial cells as workers in a human-built factory." Huiwoo Yuk (Hyunwoo Yuk), who participated in the study, believes that in the future, such living sensors can be used to create a new generation of drug delivery systems.
Below is a schematic design workflow for live material. The responses of living materials, including chemical diffusion and cell induction, are modeled in advance to provide feedback for the construction of the required sensor. The main
poloxamer involved in the printing of live sensors is
Pluronic F127 . This is a block copolymer with an average molecular weight of 13,000 Da. In dilute solutions with a polymer concentration above the critical concentration, Pluronic spontaneously forms aggregates (micelles) with a diameter of 30–50 nm with a hydrophobic central core and hydrophilic residues directed to the external environment. At a
sufficiently high concentration, micelles are in thermodynamic equilibrium with nonassociated molecules. When the solution is diluted, the micelles disintegrate, and as the surfactant concentration increases, the micelles reappear.

New technology and new modified cells made it possible to form large patterns (3 cm) with high resolution (30 microns), where cells can communicate and process signals according to specified algorithms. For professionals materials posted
here . Researchers have formed structures that are workable in terms of size and accuracy of reactions (resolution), but they also have to improve in this direction. Requires more dense patterns with lots of connections. One of the main tasks is to reproduce the architecture of a computer chip. Genetically modified bacteria will be divided into dozens and hundreds of species, with a specific reaction to specific chemicals. This will become their management system — by supplying the necessary solutions to the nodes of the three-dimensional structure, scientists will be able to start the process programmed in advance.