
Hi, Giktayms! It is time to sum up the scientific results of 2017 together with the American physical community. This time, the APS editors tried their best and prepared an extremely entertaining selection of the latest achievements of basic science. Today we will talk about them in more detail.
Gravitational-wave astronomy and all-all-all
Together with the Nobel Prize, gravitational-wave astronomy brought new surprises. The European Advanced Virgo joined the two LIGO gravity wave detectors. Now the observations of American detectors can be independently confirmed by a device of a different design, located on another continent. Moreover, the presence of three detectors allows us to determine the direction to the source of gravitational waves. I did not have to wait long: already on August 14, all three detectors recorded another signal from the merger of two black holes, the location of which (the green marker in the figure) could be determined much more accurately than with two detectors.
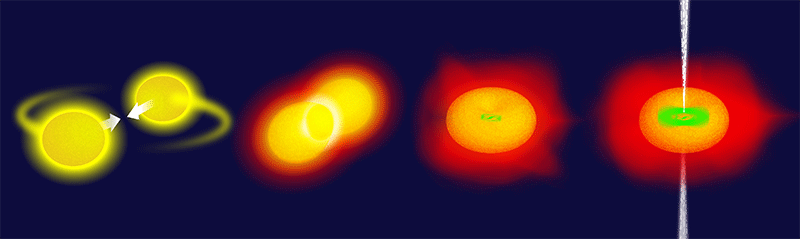
Three days later, the detectors saw a new event - this time the merger was not of black holes, but of neutron stars. By a lucky chance, at the same time with this event, a huge number of telescopes saw a flash from the merging of stars in the whole spectrum - from radio to gamma radiation. The ability to simultaneously register both light and gravitational waves is an incredible breakthrough for astronomy, which means astrophysicists will definitely not have to miss in the near future.
Cooking time crystal
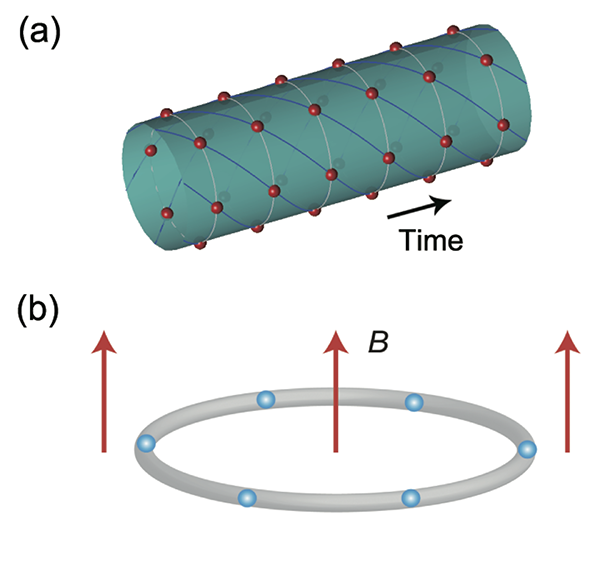
There is such a fundamental phenomenon in physics as spontaneous symmetry breaking : it occurs when the ground energy state of a system loses the symmetry inherent in the equations describing it. The most obvious example is a crystal: it turns an ordinary space, all points of which are the same among themselves, into a structure with a strictly specified period. To put it a little more scientifically, the crystal breaks the continuous translational symmetry of space, making it discrete. Since space and time are entities of the same kind, the question arises: is it possible to create a similar crystal for time - that is, to make the ground state of the system not stationary, but change periodically? Intuition suggests that there is not: a changing system usually has a non-zero kinetic energy, and therefore, is not in the ground energy state. However, in 2012, it was shown that if a system pulse is nonlinearly dependent on speed, then this becomes possible. Soon this conclusion was generalized to the case of quantum systems.

Later it became clear that, in thermal equilibrium, time crystals could not exist. However, if an external periodic effect is exerted on the system, it becomes real to create a discrete time crystal - it also periodically changes its state, but does so by a factor of several times slower than external perturbation. In other words, if the response of a time crystal is decomposed into a Fourier series, then we will see a signal on one of the subharmonics of external influence. Last year, the experimental observation of this published already two teams. The collaboration from Maryland and Berkeley used a chain of ytterbium ions for this, periodically acting on the atomic spins using laser pulses with a period T. In the intervals between pulses, the ions interacted with each other in such a way that the evolution of the entire system occurred with a period of 2T . This was the main evidence of the formation of the crystal of time. Only a month later, a group from Harvard reported a similar experiment with an ensemble of NV-centers in diamond, whose backs were excited by microwave impulses. Here the authors managed to observe oscillations with both a doubled and a tripled period. In addition to their fundamental significance, these works open up new possibilities for studying the dynamics of quantum systems, and may also be interesting for the storage of quantum states.
Causality in the quantum world
If two phenomena correlate with each other, then one may be the cause of the other. Or maybe not. Let's say there is a definite correlation between the number of tsunamis in Japan and Chile; neither of them affects the other, because both of them have a completely different root cause - earthquakes in the Pacific Ocean. In questions of the causality of correlated phenomena, the Reichenbach principle sometimes helps to understand: if it is known that the root cause of two phenomena has come, the correlation between them disappears.
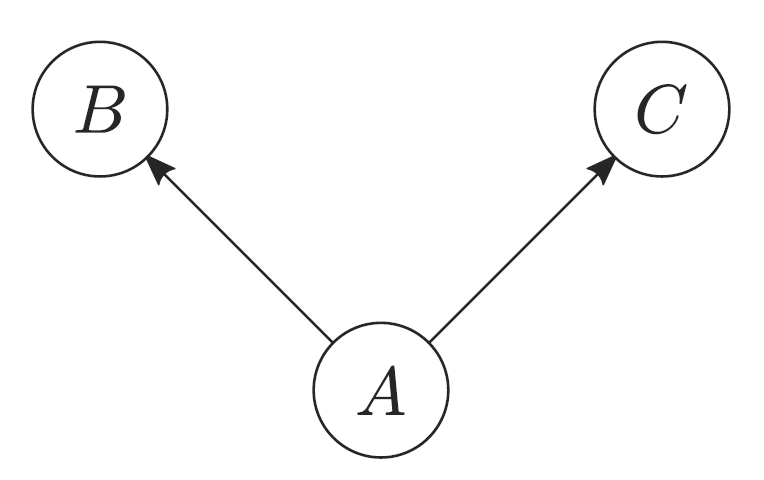
The quantum world is much more complicated. The root cause of many phenomena (for example, correlations of entangled particles) was searched for a long time in hidden parameters inaccessible to the observer. However, experiments to study Bell's inequalities showed that there are no hidden parameters (at least in any of the known species). Therefore, in the quantum world, the question itself is organized differently: not what is the cause , but what is such a quantum causality . Progress on this issue has been made by the collaboration from Britain and Canada. The authors proposed to redefine the principle of Reichenbach, moving from a deterministic classical evolution to a unitary evolution, which obey quantum systems. As a result, the first noncontradictory model was obtained, capable of quite rigorously describing quantum causality. Despite its mathematical nature, this work sheds light on the nature of quantum correlations and, possibly, will provide an opportunity to visualize quantum phenomena in a cause-and-effect language.
Wi-Fi: radar that is always with you
The idea to use the radiation of a Wi-Fi module for the radar of nearby objects is not new (for example, the work of 2005). In practice, everything is complicated by the fundamental features of Wi-Fi transmitters. First of all, they, unlike radars, radiate in all directions. This generates multiple reflections from surrounding objects and greatly complicates signal analysis. In principle, the task could be simplified by sending short pulses - but this is difficult because of the narrowband Wi-Fi.
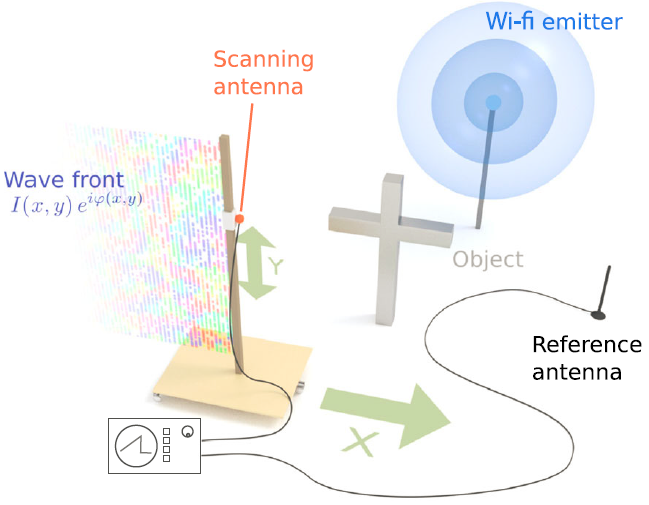
An original solution to the problem was proposed by a group from the Technical University of Munich. They record the wave front behind the object under study, and then reconstruct its shape using well-known algorithms for optical holography. In the experiment, the resolution was about 3 cm for a Wi-Fi router at a frequency of 5 GHz. A nice bonus is the fact that the source can transmit any signal - the reconstruction will work in any case. Of the difficulties - recording of the wave front has to be done pixel-by-pixel, physically moving the receiver. Using an array of receivers would greatly simplify this process by raising the frame rate to 10 fps.
Cuprate superconductors
The most high-temperature superconductors are still cuprates - compounds that include copper oxide, such as YBaCuO. Record holders go into the superconducting state already at 134 K (–139 º), while the nature of this superconductivity is still questionable. In any case, it was believed that it is not described by the BCS theory, which has worked well with many other superconductors (they are also called type II superconductors). In particular, the BCS theory predicts the existence of Abrikosov vortices , along the contour of which a continuous current flows, while inside the vortex the superconductivity disappears. Such vortices appear in a magnetic field that cannot exist in a superconductor, but easily penetrates into a non-superconducting vortex. Experimentally, Abrikosov vortices are perfectly observed in type II superconductors (confirming the BCS theory), and have never been seen in cuprates.
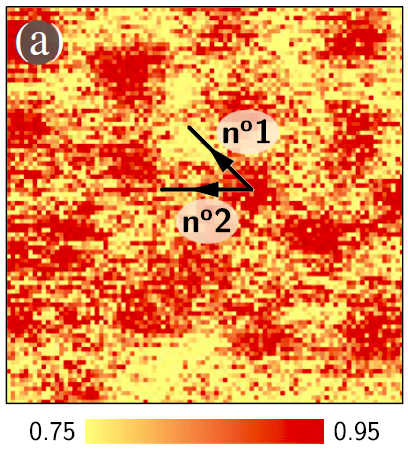
Actually, they were not seen until this year. The collaboration from Switzerland and Germany for the first time demonstrated the appearance of superconducting vortices in cuprate Y123. To do this, the authors used a scanning tunneling microscope, with the help of which they measured the conductivity of the sample over an area of 90x90 nm 2 and found an ordered vortex lattice (in the figure). Despite a number of experimental difficulties and ambiguities (mainly due to the contribution of the signal from non-superconducting electrons), the observed properties of these vortices are well described by the BCS theory, which can shed light on the nature of high-temperature superconductivity. Moreover, the approach itself, which takes into account the contribution of non-superconducting electrons to the total signal, will be crucial for future research.
The contribution of gluons to the proton spin
COMPASS spectrometer at CERN, which measured the quark contribution to the proton spin. Picture from here .
The nuclei of atoms consists of protons and neutrons, each of which, in turn, is composed of three quarks. Protons have a spin (intrinsic magnetic moment) of ½; exactly the same spin on quarks. All the more surprising are the results of experiments showing that the total spin of the proton is only 30% determined by the spin of the quarks. The reasons for this remain not entirely clear, as well as the nature of the remaining back; at the same time there are enough candidates - these are virtual quark-antiquark pairs, and the orbital angular momentum of particles, and, of course, gluons are carriers of a strong interaction that holds quarks together.

This year, a collaboration from four American universities for the first time calculated the contribution of the spin from gluons. This is done with the help of complex numerical simulation of quantum chromodynamics on a space-time lattice. It turned out that the total gluon spin is 0.25 ± 0.05 - in other words, gluons determine almost half the spin of the proton! A much smaller contribution from quarks is apparently caused by the transfer of the angular momentum to the quarks to a cloud of virtual quark-antiquark pairs and pions; The role of gluons in this process turned out to be insignificant. In general, these calculations made it possible to better understand the internal structure of the proton, and their experimental confirmation is planned to be carried out at the future American electron-ion collider.
In search of dark matter
As you know, a negative electrode is also an electrode, and a negative result is also a result. Over the past 16 months, the three largest dark matter detectors (Italian XENON1T, Chinese PandaX-II, and American LUX) have not been able to detect any traces of wimps — particles that supposedly constitute dark matter. This unequivocally shows that the existing theoretical ideas about wimps are still far from reality. Given the failure to search for supersymmetry at the LHC, someone even puts the existence of these hypothetical particles into question.

The essence of the experiments on the search for WIMPs is quite simple: the role of their detectors are huge tanks with liquid xenon, which are located deep underground, protecting against cosmic radiation. The interaction of a heavy Wimp with an xenon atom leads to a flash of light and electron generation, which are recorded by photomultipliers above and below the capacitance. Knowing the theoretical limits on the energy of wimps, one can estimate the expected number of events per unit time. The fact that such events were registered too little means that the properties of wimps are very different from the predicted ones. Apparently, if WIMPs exist, they have a different mass or a different cross section for scattering on atoms (and maybe both), which means that new generations of detectors will be required to search for them.
Machine learning recognizes topological states
Topological effects in physics are a highly topical issue that is incredibly difficult to explain on the fingers. That is why it is practically not covered in popular science literature (and this despite the tremendous successes - to recall at least about graphene, the quantum Hall effect or the Nobel Prize of 2016). In a nutshell, different topological states cannot be transformed into each other by a smooth continuous measurement of the system, which makes them extremely stable against external disturbances. The simplest example is a two-dimensional lattice of atoms whose spins either form or do not form a vortex:
Picture from here
Mathematically, these states differ in the topological charge - in this case, the number of vortices in the system with a plus sign, if the vortices are twisted clockwise, and minus - counterclockwise. In the left picture, the charge is 0, and on the right - -1. If the topological charges are different, then the states cannot smoothly transform into each other. The difficulty is that it is very difficult to calculate the topological charge. For example, if the dimensions of the vortex are huge, and it is twisted somewhere on the boundaries, then in order to calculate the charge, all the atoms in the system will have to be investigated. But there are topological charges that are much more difficult to calculate, making the calculations of new topological materials almost unaffordable.

A solution to this problem was proposed by theorists from Cornell and the University of California. Its essence is that, on the basis of the crystal lattice under study (more precisely, its electronic density), a multidimensional array (QLT image) of special integrals over contours of increasing size is generated. This allows you to cover the area of the lattice, sufficient for awareness of the topological properties. After that, a multidimensional array is fed to the input of a previously trained single-layer neural network, which concludes whether the state is topological or not. Compared with traditional methods, this method has proven to be very productive, and the authors plan to develop applications of machine learning to condensed matter physics.