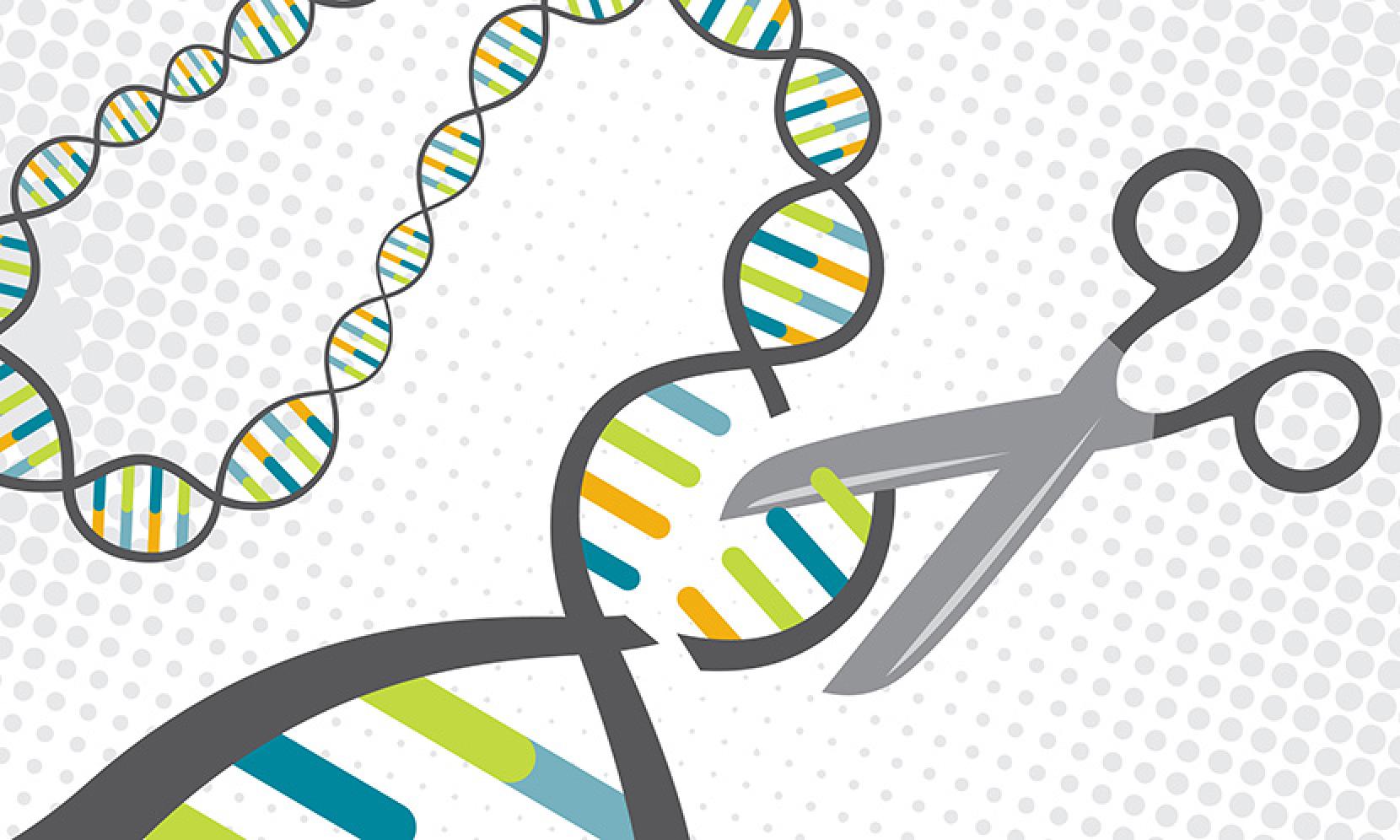
In less than five years, the technology of editing genes, known as Crispr, revolutionized modern biology. Since 2012, when its ability to find, remove, and replace genetic material was registered for the first time, scientists have published over 5,000 papers mentioning Crispr. Researchers in the field of biomedicine master it in order to better model various diseases. And countless companies began to try to extract commercial benefits through new drugs, treatments, food, chemicals and materials based on this technology.
Usually, when we refer to Crispr, we mean Crispr / Cas9 - a riboprotein complex consisting of a short strand of RNA and an enzyme that cuts DNA. He did for biology and medicine what the Model T did for production and transport — in the process of democratizing access to revolutionary technology and violating the status quo (this is a car from Henry Ford, also known as Tin Lizzy, the first in the world of the car, which was produced in millions in series from 1908 to 1927. It became a symbol of how Ford "put America on the wheels", making the car relatively affordable for a middle-class American (note M.K.).
Crispr is already being used to improve the condition of cancer patients, and next year it may be admitted to clinical trials for the treatment of genetic diseases such as sickle cell anemia and beta-thalassemia.
But, like the Model T, the Crispr Classic is somewhat awkward, unreliable and a bit dangerous. It cannot simply associate with any place in the genome. Sometimes he corrects in the wrong place. And he does not have a switch. If the "Model T" was prone to overheating, the Crispr Classic is prone to "overeating."
Even with these limitations, the Crispr Classic will remain the workhorse for science in 2018 and later. But this year, new, brighter tools for editing genes began to be released on the production line, promising to outshine their first-generation brother. So if you are just starting to think about Crispr, clip on, because genetic editing 2.0 is already here.
The targeted cutting effect of the Crispr is its defining feature. But when Cas9 cuts off two strands of DNA in the body, an element of risk is introduced - when such a sudden injury to the genome is restored, the cells may start to make mistakes. That is why scientists are developing ways to achieve the same results in a safer way.
One approach is to create a mutation of the Cas9 enzyme - such that it can still bind to DNA, but that its scissors do not work. Then, other proteins that activate gene expression can be combined with this Cas9, allowing them to turn genes on and off (sometimes with light or chemical signals) without changing the DNA sequence. Such “epigenetic editing” can be used to solve situations that arise when there are a combination of genetic factors - as opposed to simple single disorders most suitable for Crispr Classic (earlier this month, researchers from the Salk Institute used one such system to treat several diseases in mice, including diabetes, acute renal failure and muscular dystrophy).
Other scientists at Harvard and the Brodsky Institute are working on even more bold tuning of the Crispr system: editing individual base pairs, one at a time. To do this, they had to develop a completely new enzyme - not taken from natural - that could chemically convert a pair of AT nucleotides into GC. This is a small change with potentially huge consequences. David Liu, a Harvard chemist whose lab did this work, estimated that about half of the 32,000 known pathogenic point mutations in humans can be corrected by this single transformation.
“I don’t want the public to come up with the mistaken idea that we can replace any piece of DNA with any other piece of DNA in any person or any animal, or even any cell in a cup,” says Liu. - “But finding even where we are now imposes a great responsibility. The big question is how much more effective will this approach become with time? And how quickly can we translate these technological advances to the benefit of society? ”
Crispr has evolved in bacteria as a primitive defense mechanism. His task was to find enemy viral DNA and cut it until it was left. It is an accelerator without brakes, and this can make it dangerous, especially for clinical applications. The longer the Crispr stays in the cell, the higher the chance that he will find something similar to his target gene and make the cut.
To minimize these "non-target" effects, scientists have developed a number of new tools to more tightly control the activity of the Crispr.
At the moment, researchers have identified 21 unique families of natural Crispr proteins - small molecules that disable the genetic editor. But they know how only some of them work: some bind directly to Cas9, not allowing it to attach to DNA; others include enzymes that displace Cas9 for space on the genome. Researchers at the University of California at Berkeley, UCSF, Harvard, Brod, and the University of Toronto are currently working hard to turn these natural "switches" into those that can be programmed.
In addition to medical applications, this will be crucial for the further development of gene drives - gene editing technology that can quickly spread the desired modification in the population.
The ability to push evolution in one way or another will become a powerful tool for dealing with many problems, from diseases to climate change. It is considered as a tool for the destruction of anopheles mosquitoes and the extermination of other harmful species. But released, it can go out of control and, possibly, lead to dire consequences. This year alone, Darpa has invested $ 65 million in searching for safer gene drives, incl. "Switches" anti-crispr.
Despite years of success, scientists still do not understand much about how DNA errors can cause illness in humans. They know which genes are involved in cellular “guides to action”, but it’s hard to understand where these commands are delivered and how they are translated (including incorrectly) in the process. That is why the groups at Harvard and the Brodsky Institute, led by their respective Crispr Feng Zhang, are working with a new class of Cas enzymes that target RNA instead of DNA.
Because they are instructions that cell mechanisms use to create proteins, they carry more information about the genetic bases of specific diseases. And since RNA comes and goes, making changes to it will be useful for treating short-term problems, such as acute inflammation or injury. The system, which they call Repair (which stands for “RNA Editing for Programmable A to I Replacement” - “RNA editing for programmable replacement of A by I”), while it only works to convert one nucleotide. The next step is to figure out how to create the remaining 11 possible combinations.
Scientists are constantly finding new Cas enzymes. Teams at the Brodsky Institute are also working to describe the cpf1 version of Cas, which leaves sticky ends instead of being dephosphorylated when cutting DNA. In February, a group from UC Berkeley discovered CasY and CasX, the most compact Crispr systems. And researchers expect that many others will appear in the coming months and years.
Only time will tell whether Crispr-Cas9 was the best of them, or simply the first to capture the minds of a generation of scientists. “We don’t know what will work best in different applications,” says Megan Hochstrasser, who made her Ph.D. in the laboratory of the Crispr companion Jennifer Dudna and now works in the Innovation Institute of Genomics. “So at the moment I think that everyone needs to bet on all these tools at the same time.”
It will take many years of work for the current generation of gene editors to move from the lab to real patients, lines of vegetables, and agricultural pests carrying diseases.
If genetic editing 3.0 will not make them obsolete first.